This blog-post from Martin Persson at Chalmers University of Technology in Sweden takes on the timely question of how one should measure the climate impacts of different greenhouse gases. He begins by explaining what the two most common metrics - Global Warming Potential (GWP) and the Global Temperature change Potential (GTP) – actually measure. He then moves on to focus specifically on beef and associated methane emissions. In light of the Paris Agreement’s target of limiting warming to 2oC he argues that the ‘right’ metric to choose needs to reflect the specific climate policy that the measurement is to serve.
Martin Persson is an Associate Professor at Physical Resource Theory, Department of Energy & Environment at Chalmers University of Technology in Sweden. In addition to metrics, his research focuses on global land use in relation to climate, biodiversity, and conservation policy. In a recent paper he and his colleagues show that the consumption and international trade of beef, soy, palm oil and timber products are dominant causes of deforestation in the tropics.
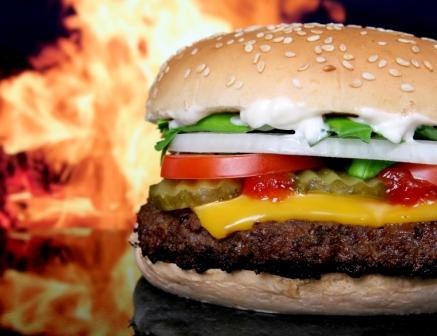
How do you compare the climate impact of, say, eating a hamburger and driving your car to the local supermarket? Making this comparison requires a conversion factor—a greenhouse gas metric—for adding up the emissions of different gases resulting from these different activities: carbon dioxide from the combustion of fossil fuels, methane from enteric fermentation and manure and nitrous oxide from feed production. The metric most commonly employed in these type of calculations are Global Warming Potentials (GWPs), evaluated over a time horizon of 100 years, according to which a ton of methane and nitrous oxide, respectively, equals about 30 and 300 tonnes of carbon dioxide.
But GWPs are not the only possible way to convert methane and nitrous oxide emissions to carbon dioxide-equivalents. Another option is to use so called Global Temperature change Potentials (GTPs), according to which methane is just four times stronger than carbon dioxide as a greenhouse gas (if adopting the same, 100 year, time horizon). Not surprisingly, given the high methane emissions associated with beef and dairy production, using GTPs instead of GWPs substantially lowers the carbon footprint of these products (especially under optimistic scenarios regarding carbon sequestration in pastures).
So, how do you decide on which metric to choose? Answering that requires an understanding of what these different metrics actually seek to equate.
GWPs focus on radiative forcing, a measure of the radiative energy imbalance due to increased levels of the greenhouse gases that cause the atmosphere, land and oceans to warm. To calculate the GWP of a gas, you add up the total (cumulative) radiative forcing resulting from the emission of one tonne of the gas today over a given time horizon, and then compare that to the total radiative forcing over the same time horizon resulting from emitting one tonne of carbon dioxide. GTPs, on the other hand, compare the temperature change at a given point in the future, resulting from an emission of one tonne of a gas today, and compare that to the temperature rise at the same point in time from emitting a tonne of carbon dioxide today. The calculations of both these metrics are illustrated graphically in the figure below (note the differences in scale between the two graphs).
The graphs shows the radiative forcing (measured in mW/m2; blue lines) and temperature change (measured in mK; red lines) resulting from the emission in year 0 of one million tonnes of carbon dioxide (left panel) and methane (right panel) (note the differences in scale between the two graphs). The Global Warming Potential (GWP) value for methane is calculated by integrating the radiative forcing over a given time period, usually 100 years (light blue shaded area in right graph), and dividing this by the integrated radiative forcing for carbon dioxide over the same time period (light blue shaded area in left graph). Methane’s Global Temperature change Potential (GTP) is calculated by dividing the temperature change at a given point in time resulting from the methane emission at year 0 by the corresponding temperature change for carbon dioxide (for 100 year time horizon, represented by the red triangles and call-outs in both panels).
The figure above clearly shows that due to the differences in atmospheric lifetimes of carbon dioxide and methane, the temporal dynamics of the radiative forcing and warming arising from emissions of the two gases are markedly different. Methane’s short atmospheric lifetime—about 12 years—implies that much of the temperature perturbation resulting from an emission today will have dissipated in 100 years’ time (but not all, as thermal inertia implies that it takes time for the world’s oceans both to heat up and cool down). Consequently, for longer time horizons the GTP value for methane will be much lower than the corresponding GWP value, since the latter reflects the cumulative warming effect of an emission and hence also accounts for the near-term climate impacts caused by methane emissions (and not just the change lingering at the end of the period). Thus, although emitting 30 tonnes of carbon dioxide or 1 tonne methane today (i.e. equal emissions if weighted by the 100 year GWP) will have the same impact on total radiative forcing over the coming century, it will give drastically different absolute temperature changes at the end of this period (about seven times higher for carbon dioxide than for methane, as methane’s GWP value is about seven times higher than its GTP value).
This example illustrates a general insight when it comes to greenhouse gas metrics: because of the differences in the atmospheric lifetime across greenhouse gases, there can never be a perfect metric that assures equivalence across all relevant impacts of climate change. Consequently, the choice of metric will always reflect value judgments, such as: (1) which impact to compare (e.g., radiative forcing, temperature change or sea-level rise); (2) whether to compare these impacts at some future date or cumulative impacts over the whole period up until this date; and (3) time-horizon over which impacts are assessed.
This does not, however, imply that the choice of metric is arbitrary. A common argument is that the choice of metric must reflect the climate policy goal the metric is to serve. For instance, cumulative radiative forcing, as measured by GWPs, relates directly to cumulative warming, which is a crude proxy for climate damages. Hence, if our policy goal is to limit the total amount of climate damages over some specified time period, GWPs would be a good metric to use. However, if we are simply interested in staying below some climate threshold—e.g., keeping warming well below 2oC, as stipulated by the Paris Agreement of last year—and do not care about the path leading up to this target, we might favour GTPs. This is because for a distant temperature target, it only matters how much warming is left lingering from an emission today at the point when the temperature target is reached (and not how much warming we get leading up to this point).
Note, though, that if this is the argument for choosing GTPs over GWPs, one must also be aware of other implications of this choice. First, it is not reasonable to adopt a 100 year time horizon in GTP calculations. Instead, the time horizon should reflect the time remaining until the temperature target is reached. For the 2oC target this is likely to happen sometime between 2050-2100. We have shown in a recent paper that if 40-90 years is a reasonable time horizon to use in GTP calculations, the resulting GTP value for methane today is 18, not four. This is because the temperature impact of releasing a tonne of methane today increases rapidly the closer the point in time it is evaluated (see the figure above).
Second, it does not make sense to adopt a constant time horizon for GTPs. (Why should we always be interested in the warming happening at some distant point in the future?) Instead, as we approach the temperature target the time horizon over which we evaluate GTPs should decrease, resulting in a valuation of methane that rise rapidly (since the short-term warming effects become increasingly important), reaching a value of 120 when the target is met.
Consequently, the impact of switching from GWPs to GTPs would have a modest impact on the carbon footprint of beef today: a reduction from 23.5 to 18.9 kg CO2-equivalents per kg carcass weight, for average EU beef production. By mid-century, however, the carbon footprint calculated using GTPs could potentially rise to 63 kgCO2-eq./kg beef, if it turns out that by then 2oC warming is imminent. The choice of metric, thus, will have a large impact on the future role of the livestock sector in climate mitigation (more so than in the present).
Finally, the discussion on metrics may partly obscure a key difference between emissions of fossil carbon dioxide and other, more short-lived, greenhouse gases: while emissions of the latter will eventually be completely broken down and removed from the atmosphere, part of our carbon dioxide emissions (20-30%) will stay in the atmosphere for more than thousands of years. The practical implication of the latter is straightforward: the only way to stabilize carbon dioxide concentrations in the atmosphere is to bring down emissions close to zero. This is why we talk about a finite carbon budget; a target for cumulative carbon emissions we cannot exceed if we are to limit warming to 2oC.
For other greenhouse gases, however, it is enough to stabilize emissions in order to stabilize atmospheric concentrations (though the higher the emissions, and the longer the lifetime of the gas, the higher the resulting concentration). As a result, one can compare the long-term climate impacts of an emission pulse of carbon dioxide, with a constant emission rate for more short-lived greenhouse gases. Put differently, reducing annual emissions of methane and nitrous oxide will relax the carbon budget compatible with a 2oC target. Or, conversely, for every tonne of carbon dioxide emissions mitigated, we can increase annual emissions of more short-lived greenhouse gases forever without affecting long-term warming. For methane, emitting one kg per year has the same long-term temperature impact as would a one-time emission of around 5 tonnes of carbon dioxide. For nitrous oxide, which is both a more potent greenhouse gas and has a much longer atmospheric lifetime, an annual emission of one kg compares to a one-time emission of about 100 tonnes of carbon dioxide (see the figure below).
This graph shows the radiative forcing effect over 1 000 years from either emitting one tonne of nitrous oxide or 27 tonnes of methane every year, or emitting 120 000 tons of carbon dioxide in year zero only. As some of the carbon dioxide emitted remains in the atmosphere for thousands of years, the long-term impact of a pulse emission will have the same long-term effect on the earth’s radiative balance as that of annual emissions of the other gases, which are constantly broken down and removed from the atmosphere.
Using these numbers, the annual emissions from the global livestock sector (some 112 million tonnes of methane and 8 million tonnes of nitrous oxide), if held constant, have the same long-term climate impact as carbon dioxide emissions of roughly 1 000 billion tonnes. This is in the same order of magnitude as the total remaining carbon budget under a 2oC target! Seen this way, achieving significant cuts in the global greenhouse gas emissions from the livestock sector—through productivity increases, technological development, and dietary changes—can substantially raise the carbon budget compatible with the climate target currently agreed by the global community, hence increasing the likelihood that this target will actually be met.
Post a new comment »